Competing ideas abound for how Earth got its moon
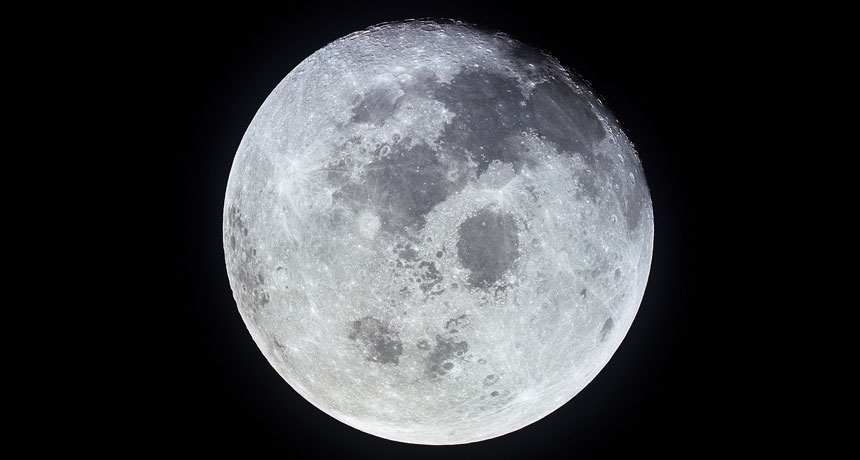
The moon’s origin story does not add up. Most scientists think that the moon formed in the earliest days of the solar system, around 4.5 billion years ago, when a Mars-sized protoplanet called Theia whacked into the young Earth. The collision sent debris from both worlds hurling into orbit, where the rubble eventually mingled and combined to form the moon.
If that happened, scientists expect that Theia’s contribution would give the moon a different composition from Earth’s. Yet studies of lunar rocks show that Earth and its moon are compositionally identical. That fact throws a wrench into the planet-on-planet impact narrative.
Researchers have been exploring other scenarios. Maybe the Theia impact never happened (there’s no direct evidence that the budding planet ever existed). Instead of a single colossal collision, scientists have proposed that a string of impacts created miniature moons largely from terrestrial material. Those mini moons merged over time to form one big moon.
“Multiple impacts just make more sense,” says planetary scientist Raluca Rufu of the Weizmann Institute of Science in Rehovot, Israel. “You don’t need this one special impactor to form the moon.”
But Theia shouldn’t be left on the cutting room floor just yet. Earth and Theia were built largely from the same kind of material, new research suggests, and so had similar compositions. There is no sign of “other” material on the moon, this perspective holds, because nothing about Theia was different.
“I’m absolutely on the fence between these two opposing ideas,” says UCLA cosmochemist Edward Young. Determining which story is correct is going to take more research. But the answer will offer profound insights into the evolution of the early solar system, Young says.
The moon is an oddball. Most of the solar system’s moons are way out among the gas giant planets. The only other terrestrial planet with orbiting satellites is Mars. Its moons, Phobos and Deimos, are small, and the prevailing explanation says they were probably asteroids captured by the Red Planet’s gravity. Earth’s moon is too big for that scenario. If the moon had come in from elsewhere, asteroid-like, it would probably have crashed into Earth or pulled off into space. An alternate explanation dating from the 1800s suggested that moon-forming material flew off of a fast-spinning young Earth like children tossed from an out-of-control merry-go-round. That idea fell out of favor, though, when scientists calculated that the spin speeds required were impossibly fast.
In the mid-1970s, planetary scientists proposed the giant-impact hypothesis and the mysterious planet-sized impactor (named Theia in 2000 for the Greek deity who was mother of the moon goddess Selene). The notion made sense given that the early solar system was like a game of cosmic billiards, with giant space rocks frequently colliding.
A 2001 study of lunar rocks collected during the Apollo missions cast doubt on the giant-impact hypothesis. The research showed that the Earth and moon had surprising similarities. To determine a rock’s origin, scientists measure the relative abundance of oxygen isotopes, which act something like finger-prints at a crime scene. Rocks from Earth and its moon, the scientists found, had seemingly identical mixes of oxygen isotopes. That didn’t make sense if much of the moon’s material came from Theia, not Earth. Using impact simulations, Rufu and colleagues recently estimated that the chance of a Theia collision yielding an Earthlike lunar composition is very slim.
Studies of other elements in Apollo rocks, such as titanium and zirconium, also suggest that the Earth and moon originated from the same material. Young and colleagues recently repeated the oxygen isotope measurements with the latest techniques, hunting for even the slightest difference between Earth and the moon. In January 2016, the team published the results in Science. “We measured the oxygen to the highest precision available,” Young says, “and, gosh, the Earth and moon still look identical.”
Some scientists have built simulations of a giant Theia impact that fashion a moon made mostly from terrestrial material. But the scenarios struggle to match the modern positions and movements of the Earth-moon system.
It’s time to think outside the giant-impact box, some scientists argue. Not one but many impacts contributed to the moon’s formation, Rufu and colleagues proposed January 9 in Nature Geoscience. The moon, they say, has an Earthlike composition because most of the material flung into orbit from these impacts came from Earth.
Mini-moon merger
The multi-impact hypothesis was first put forward in 1989, though scientists at the time didn’t have the computer power to run the simulations that could support it. Rufu and colleagues recently revisited the proposal with computer simulations of multiple impactors, each about a hundredth to a tenth of Earth’s mass, smacking into the early Earth.
Any impactors that were direct hits would have transferred lots of energy into the Earth, excavating terrestrial material into space. Debris from each impact combined over centuries to form a small moon, the simulations show. As more impacts rocked Earth over tens of millions of years, more moons formed. Gravity pulled the moons together, combining them. Over roughly 100 million years, according to this scenario, around 20 mini moons ultimately merged to form one mighty moon (SN Online: 1/9/17).
The multimoon explanation yields the right lunar mix in simulations roughly 20 percent of the time, better than the 1 to 2 percent for the giant-impact hypothesis, the researchers note. “The biggest takeaway is that you cannot explain everything with one shot,” Rufu says.
Planetary scientist Robin Canup finds the scenario convincing. “To me, this appears to be a real contender alongside the one big impactor hypothesis,” says Canup, of the Southwest Research Institute in Boulder, Colo.
Don’t discount Theia
But the Theia hypothesis has recently found fresh support. The odds of Theia resembling Earth’s composition enough to yield an Earthlike moon may be a lot higher than originally thought, new chemical analyses suggest. Most of the material that makes up Earth came from the same source as a type of meteorite called enstatite chondrites, planetary scientist Nicolas Dauphas of the University of Chicago reported January 26 in Nature.
Just as with oxygen, the isotopic mix of various other elements in Earth’s rocks serves as a fingerprint of the rocks’ origins. Some of these elements are iron-lovers, such as ruthenium, which quickly sink toward Earth’s iron-rich core (SN: 8/6/16, p. 22). Any ruthenium found close to Earth’s surface, in the mantle, probably arrived late in Earth’s development. Iron-indifferent elements like calcium and titanium don’t sink to the core; they stay in the mantle. Their isotopes record what went into Earth’s assembly over a much longer period of time. By looking at the iron-lovers and iron-indifferent elements together, Dauphas created a timeline of what types of space rocks added to Earth’s mass and when.
A mix of different rocks, including some resembling enstatite chondrite meteorites, supplied the first 60 percent of Earth’s mass, Dauphas says. The remaining balance came almost exclusively from the meteorites’ precursors. In total, around three-quarters of Earth’s mass came from the same material as enstatite chondrites, Dauphas estimates. If Theia formed at around the same distance from the sun as Earth, then it primarily formed from the same material, and consequently had a similar isotopic composition. So if the moon formed largely from Theia, it makes sense that lunar rocks would have a similar composition to Earth, too.
“Most of the problem is solved, in my opinion, if you admit that the great impactor’s material was no different than that of the [early] Earth,” says cosmochemist Marc Javoy at the Institute of Earth Physics of Paris. “It’s the simplest hypothesis” and would mean that the material gobbled up by budding planets in the inner solar system was fairly uniform in composition, offering insight into the arrangement of material that built the solar system.
The notion that Earth is made from the same material as enstatite chondrites “doesn’t make many people happy,” says geochemist Richard Carlson of the Carnegie Institution for Science in Washington, D.C. The isotopes in Earth’s mantle and the meteorites may match, but the relative abundance of the elements themselves do not, Carlson wrote in a commentary in the Jan. 26 Nature. An additional step in the process is needed to explain this compositional mismatch, he says, such as some of the element silicon getting stashed away in Earth’s core.
“What we have now are a lot of new ideas, and now we need to test them,” says Sarah Stewart, a planetary scientist at the University of California, Davis.
One recently proposed test for the moon’s formation is based on temperature, though it seems to be consistent with both origin stories. A new study comparing the moon’s chemistry with glass forged by a nuclear blast suggests that temperatures during or just after the moon’s inception reached a sizzling 1400° Celsius. That means any plausible moon-forming scenario must involve such high temperatures, researchers reported February 8 in Science Advances.
High heat causes rocks to leach light isotopes of zinc. The green-tinged glass forged in the heat of the 1945 Trinity nuclear test in New Mexico lack light isotopes of zinc, says study coauthor and geologist James Day of the Scripps Institution of Oceanography in La Jolla, Calif. The same goes for lunar rocks. Such high temperatures during or just after the moon’s formation fit with the giant-impact hypothesis, he says. But Rufu calculates that her multi-impact hypothesis also yields high enough temperatures.
So maybe temperature can’t resolve the debate, but probing the composition of Earth and the moon’s deep interiors could prove the mini-moon explanation right, says Rufu. Without a single giant collision, the interiors of the two worlds may not have been well mixed, she predicts. Dauphas says that measuring the compositions of other planets could lend credence to his Earthlike Theia proposal. Mercury and Venus would also have formed largely from the same kind of material as Earth and therefore also have Earthlike compositions, he says. Future studies of the solar system’s inhabitants could confirm or rule out these predictions, but that will require a new chapter of exploration.